New perk! Get after it with local recommendations just for you. Discover nearby events, routes out your door, and hidden gems when you sign up for the Local Running Drop.
Power meters are nothing new in the cycling and running worlds, but when it comes to swimming with power, the sport is widely considered to be on the verge of a technological revolution. Wearable technology is getting smaller, has a longer battery life, and is increasingly waterproof. These aspects will allow triathletes and swimmers to gather advanced data to help with pacing and analyze swim mechanics in real-time and bring power-based training into the water.
Power-based training is considered one of the most accurate ways to monitor your cycling (and in running it’s been around for about a decade, but hasn’t quite caught on in the same way—yet). Unlike training using a heart-rate monitor or by perceived effort, there is very little margin for error with power. It is measured in watts and, in cycling, it is the result of the torque (force on the pedals) multiplied by your cadence (RPM, revolutions per minute). It is absolute—that is, a power meter gives you real-time, accurate data that is a reflection of the actual work being done. By comparison, your heart rate, while also a valuable data point, can be affected by a myriad of factors, such as fatigue, caffeine, stress, and other environmental factors. So how does power play out in the pool?
RELATED: Riding with a Power Meter Will Supercharge Your Training
What is Swimming with Power?
When we swim, we produce more than 80% of our propulsion with our hand and forearm. Also, the arm and shoulder are very similar to a bike crank. So, let’s start out by using cycling with power to help explain swimming with power. Cycling power is calculated as: Power = Torque x Cadence, where Torque = Force x Crank Length. We can substitute the torque equation into the power equation, and we get the complete cycling power equation: Power = Force x Crank Length x Cadence
Now, calculating power for swimming is very similar: Power = Torque x Stroke Rate, where Torque = Force x Distance from shoulder to hand
We can substitute the torque equation into the power equation, and we get the complete swimming power equation, which is: Power = Force x Distance from shoulder to hand x Stroke Rate
Another way to look at generating power while swimming is by imagining the swimmer’s arm and shoulder are a bike crank. We know that you will generate power if you press on your pedal while pedaling. Figure 1 (below) demonstrates this concept:
The basic mechanics for calculating swimming power are calculated the same way. For example, look at Figure 2 and imagine the swimmer’s shoulder is the center of the bike crank. The distance from the swimmer’s shoulder to the swimmer’s hand is the crank length, and the force the swimmer’s hand produces while moving through the water is the same as the force placed on the pedal while pedaling. This set up of the front quadrant swim mechanics allows swimming power to be calculated the same way as cycling power.
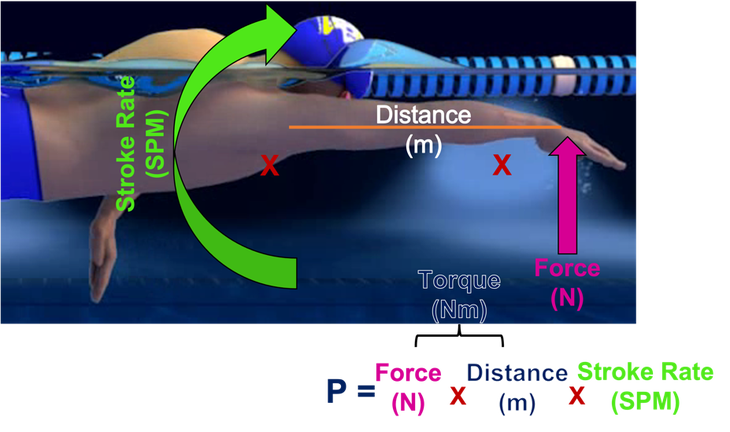
How can swimming with power improve swimming for triathlon?
Swimming with a power meter provides triathletes with a wealth of data. But, more importantly, it gives an objective pacing metric. Typically, triathletes and swimmers swim by pace. Whether the pace is a group method, such as 100 repeats on a 1:20 interval or by individual pace calculated via critical swim speed (CSS) methodology. The problem with pace is it provides a single measurement of how fast you are going over a particular distance. This aspect is not the case with power meters.
There is currently only one in-water swimming power meter, the TraineSense SmartPaddle, which has been around for about five years (you can see it in action here). It is a force meter that measures how water flows through the paddle as your hand goes through the water. This action allows the swimmer or coach to see how the athlete’s hand tracks through the water and produces force. The athlete or coach can make corrections to the athlete’s front quadrant swim stroke to improve efficiency and force output. The swimmer has more forward propulsion and becomes faster with less energy cost by improving efficiency and power. The only issue with this device is that it’s currently only available in Europe and, for the complete kit, it comes with a hefty 800 Euros price tag (~$865). If you can get your hands on one (they are currently out of stock), anyone can use it (no specialist knowledge is required), although there’s a little learning curve to analyzing its data.
Now, let’s walk through an example of using the the TraineSense SmartPaddle in the pool:
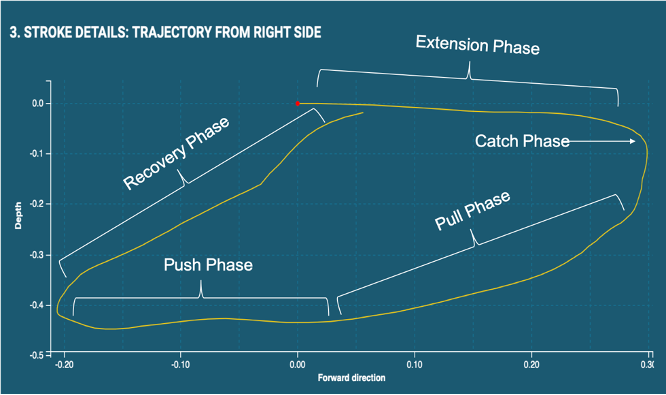
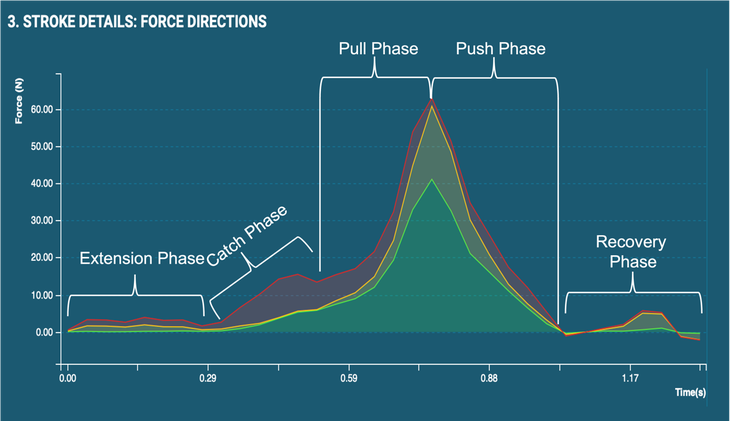
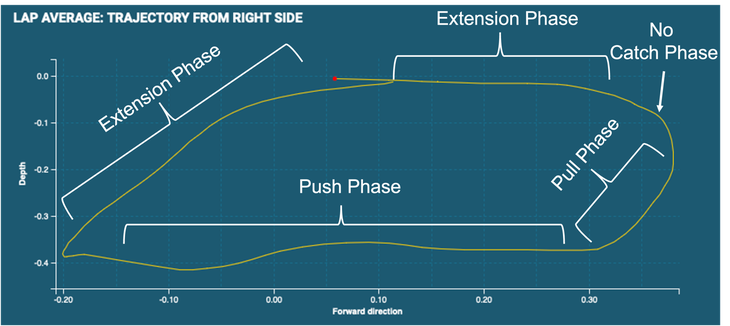
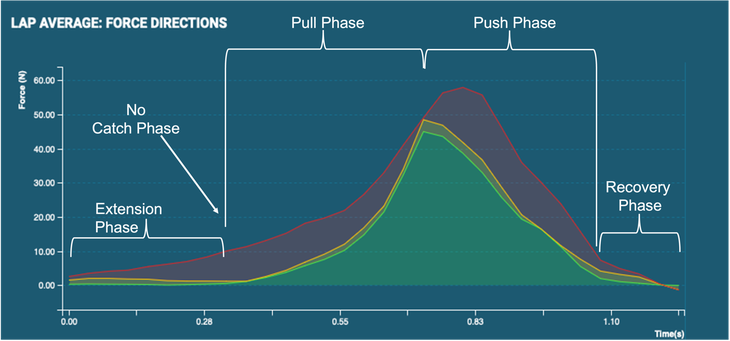
Figures 3 through 6 depict the arm trajectory and force profile of a freestyle swim stroke. Here are some details we can glean from the information gathered by a swimming power meter:
Catch phase:
In Figure 3, there is a distinct catch phase. As a result, the graph makes a divergent downward change in direction. When the force meter is placed at a different angle, which occurs with the high elbow catch, we see this marked change in direction. When the catch phase is performed correctly, the force curve has a sharp rise in force output, as seen in Figure 4.
Now, in Figure 5, we see the complete opposite. In this example, the triathlete has no catch. The trajectory is more rounded than the distinct direction change seen in Figure 3. The athlete does not perform the high elbow as the arm extends through the extension phase. Instead, the arm stays fully extended at the elbow and shoulder throughout the pull and push phases. In Figure 6, this action causes a slow rise in force production that is less than what was achieved when the stroke was correctly performed (Figure 4).
Push phase:
In Figure 5, the push phase is much more elongated than in Figure 3. This elongation occurs because the arm is fully extended; the arm travels a longer distance through the stroke cycle than when the high elbow is performed. This arm action also causes the force curve to be elongated for the push phase (Figure 6).
RELATED: The 4 Phases of the Freestyle Swim Stroke
So what does this tell us?
Figures 3 through 6 provide robust data to make high-value adjustments to the athlete’s swim stroke. Figures 5 and 6 show us how the athlete’s swim stroke changed when fatigue occurred. First, the athlete needs to work on the high elbow to produce a catch phase. By adding in the catch, the athlete has the potential to generate more force, as seen in Figure 3. Also, the high elbow will shorten the stroke length, especially the push phase, to allow for a higher stroke rate. Finally, increasing the stroke rate will increase the power output to increase forward propulsion.
Power meters are not limited to in-water training. Swimming ergometers, such as the VASA Swim Erg, exist for dryland training. These systems have power meters that measure torque on a flywheel. Due to this, we do not get the trajectory data we see in the water. However, we do get a wealth of data. Let’s walk through an example utilizing a swimming ergometer.
RELATED: How to Swim With a VASA Ergometer
In Figures 7 and 8 (below), a triathlete performed a swimming functional threshold power test (sFTP) as outlined in the book, Triathlon Training with Power. Here is some of the information gleaned from the test:
- Consistency of power output: The triathlete was not very consistent throughout the 1500m sFTP test. It is essential to note this was the triathlete’s first sFTP test on the swim ergometer. There are significant peaks and valleys which hurt the overall average power output.
- Stroke rate: The sFTP test stroke rate was in the mid-40s. This stroke rate is similar to the stroke rate the triathlete performs in the pool.
- Power balance: The triathlete has a significant imbalance in power production between the left and right arms. Figure 8 demonstrates that the triathlete produces slightly more power with the left arm than the right arm. Even though not shown, the average left and right arm power production balance was 54% left arm to 46% right arm.
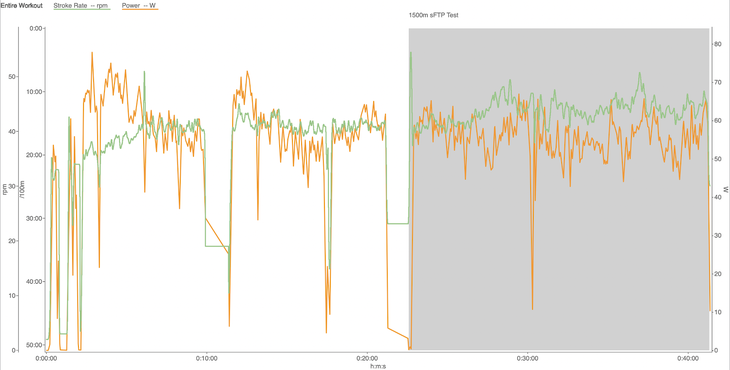
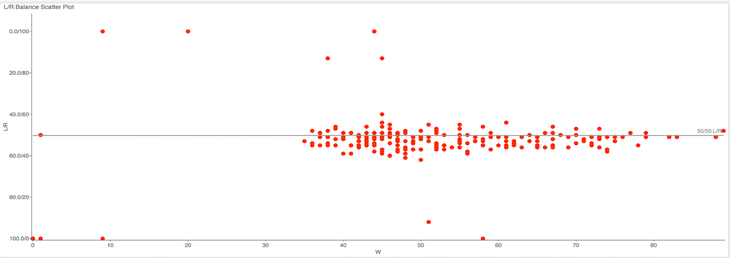
The ultimate data point gleaned from the sFTP data was the imbalance in power production. This data gives the triathlete an area of focus to improve efficiency. Furthermore, the triathlete performed a sFTP which can be used to calculate personalize power-based training zones, exactly as we would with bike FTP tests.
Is this something that’s going to catch on?
Swimming power meters are in their infancy. The technology has only been around for a few years, whereas running power meters have been around for about 10 years and cycling power meters for nearly 30 years. However, there is a limitation to swimming power meters. The most obvious is real-time data in the water. We do not have a way to see our power and force data while swimming in the water in the same way that we can while running and cycling. As technology evolves, this may soon change.
We are starting to see goggles, such as the FORM goggles, with heads-up displays (HUD), that show your swimming metrics while you swim. These devices do not currently offer power data. Yet, as the technology matures, they may be able to integrate the water-based swimming power meter data for real-time actionable data. With the swimming ergometers, we can use a smartwatch to collect the power data and see it in real-time. The triathlete can utilize the data for pacing and power-based training. Depending on the ergometer, the device can be connected to a tablet or computer and used with training software such as TrainerRoad. However, the technology is a little on the pricey side.
A VASA Swim Erg can cost close to $2,000. The TraineSense SmartPaddle, too, as outlined above, is cost-prohibitive. As more power meters come onto the market, these prices will go down. (Keep an eye out for the Zen8 system, for example). Remember that only three to five cycling power meters were on the market until about eight years ago, but there are now more than 30 different cycling power meters available. In my opinion, I believe we are about five years away from seeing a full integration of this technology in the water. It is a technology that can and will—in time—catch on and revolutionize how we approach our swim training and performance.
Chris Myers has a PhD in exercise physiology and is a USAT and Ironman-certified coach who works with triathletes of all abilities.